A Closer Look at Lenticular Clouds
By Karl Philippoff
I grew up in New Jersey, and while that was great for many reasons, one of the things my home state is missing is large mountains, both to recreate in and to see from afar. Living on top of New England’s tallest mountain for 8 days at time has served to replace this something that was missing and then some! One of the attractions of living up here is to be at an excellent viewpoint to have bird’s eye view of the clouds (when we are not in fog, of course) and how they may change during the course of a few minutes or hours. One of the neater clouds that I do not recall seeing until becoming an intern up here are lenticular clouds.
These visually striking clouds have been variously described to appear as lenses, upside-dinner plates, stacks of pancakes, almonds, or UFOs. They usually form when a relatively strong flow of stable air flows into a barrier, most commonly a mountain range, and is forced upward. As the air ascends, it expands and cools. If the air has sufficient moisture, this cooling may be enough to cause condensation to occur, and therefore generate clouds. Because the air is stable, however, it will resist this vertical motion and seek to descend to its former level. As it descends, it will contract and warm, causing the newly formed cloud to evaporate. What is unique about these clouds is that they are very dynamic, and while each individual air parcel is undergoing this cycle of cooling and condensing and warming and evaporating, visually, the cloud appears to be standing still. This is because the waves that form behind the barrier are standing waves, very similar in shape and form to what occurs as water in a river flows over objects and creates waves downstream. These are called ‘standing waves’ for this reason, in that they remain in place even as flow continues through them. These wave trains can extend for long distances downwind of the barrier, with lenticular clouds forming at the crest of each subsequent wave (See Figure 1).
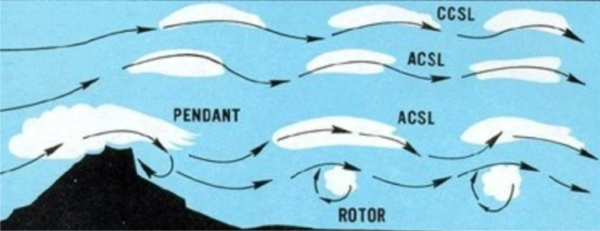
Figure 1: Lenticular cloud diagram.
The above simplified diagram represents stable atmospheric flow from left to right. As the atmosphere is forced up and over a barrier, such as a mountain, lenticular clouds may form at different elevations downwind of the barrier. ‘CCSL’ in the diagram refers to CirroCumulus Standing Lenticular clouds which form at high elevations, whereas ‘ACSL’ in the diagram refers to AltoCumulus Standing Lenticular clouds which form at middle elevations. ‘SCSL’, which do not appear on the diagram, refer to StratoCumulus Standing Lenticular clouds, which form at low elevations. The primary difference between the cloud types is the phase of the cloud, with the high clouds being composed of mostly ice crystals, and the mid-level clouds being composed of both ice crystals and liquid water droplets; the low clouds being composed of almost exclusively liquid water droplets. The phase of water in the clouds changes their morphology, allowing elevation to be estimated, as temperature typically decreases with increasing elevation.
This is the reason we code them in our observations as CCSL if they form above 18,000 feet in elevation. ACSL is the code if they form between 8,500 feet and 24,000 feet in elevation, and SCSL if they form below 8,500 feet in elevation, with the first two letters referring to the level of the cloud, and the last two letters referring to the fact that these clouds appear to be standing still.
A way to perhaps more intuitively understand the motion of the air over and beyond a barrier is as mass suspended by a spring. The stable air flowing into the barrier can be thought of as the system at rest, with a stationary mass being held by the spring and with no motion occurring. The stable air flowing into the barrier could be thought of as lifting the mass, with the spring contracting. But once the barrier has been crossed, that can be thought of letting go of the mass, descending past its original resting point and then oscillating above and below this point. Eventually, these oscillations will tend toward smaller and smaller deviations before becoming at rest once again. The reason the air will tend to oscillate around that resting point is because it was stable before it was disturbed. Stable air, by definition, resists vertical motion and will act to return to its prior state or level after being disturbed.
The spring in this analogy represents the stability profile of the atmosphere. A more stable atmosphere would be represented by a stiffer spring, and a less stable atmosphere would be represented by a looser spring. It does not mean it is immovable, however, as in this example. Since the air is flowing horizontally as these vertical motions occur, the trace of the mass as seen perpendicular to its motion would be similar to a damped sine wave, with the waves largest right when the mass is dropped, and trending toward smaller and smaller waves further from the disturbance at later times. Since air is continuously flowing over the obstacle, all of these oscillations are visible at the same time.
Using this analogy, it is easy to imagine different scenarios of the spring/mass system and what effect they might have on the oscillations. A stiffer spring (more stable atmosphere) would result in more rapid, closely spaced oscillations with little vertical movement, while a looser spring (less stable, but not unstable) would result in slower, more gradual and larger oscillations. However, using only these two components leaves out the effect of the wind speed. Higher winds would act to space out the oscillations horizontally, with higher winds corresponding to longer spaces between the crests, even if the time it took to make one full oscillation remained the same.
Unstable air accelerates upward or downward in response to a disturbance. This results in great turbulence close to the barrier or obstacle, but these eddies than quickly dissipate as mixing dissipates the turbulence. Going back to the rapids in the stream analogy, ‘stable’ flow would be similar to many non-breaking wave crests which extend a good deal downstream of the obstacle, whereas ‘unstable’ flow would be analogous to a massive breaking rapid immediately downstream, but that does not extend a great distance downstream. Thus the ‘peak’ turbulence is higher in unstable flow, but the turbulence at some distance from the barrier can be higher when the air is stable. Also, it is important to note that at large scales, the atmosphere as a whole is usually neutral to stable and is very rarely unstable to any great depth.
Well, I did say that they look very cool. Let’s look at some pictures and some time-lapse photography taken of lenticular clouds taken from on or around the summit. In the simplest case, here are a few photographs of single-layer lenticular clouds. They can be isolated, appearing like relatively small flattened disc (Image 1), larger sheet-like forms (Image 2), longitudinally extended arcs (Image 3). They also can seem to form groups located at several different layers with layers loosely grouped (Image 4), or, much more often stacked closely together at different levels. In Image 5, the upward motion beneath the stack of lenticulars is forming a rotary cloud.
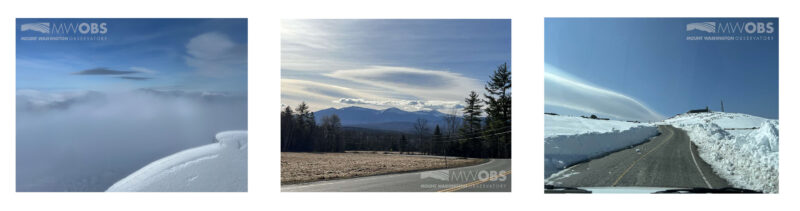
Images 1-3 (left to right).
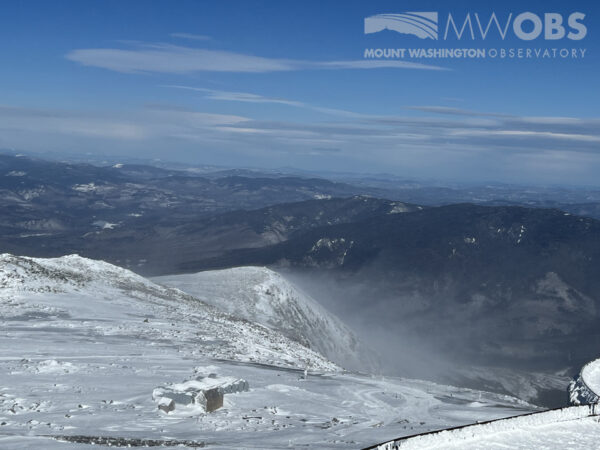
Image 4
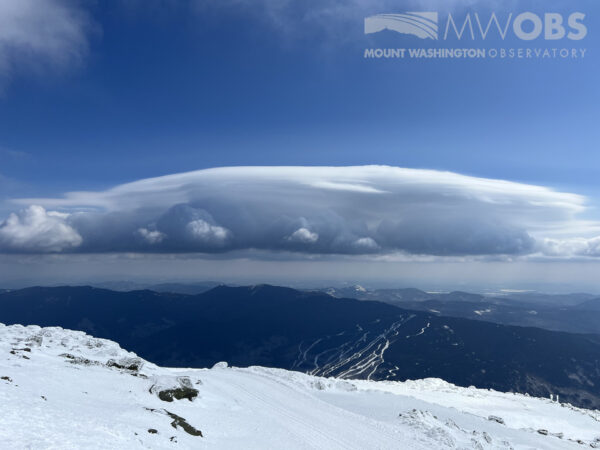
Image 5
Pictures can tell you a lot about their shape, but to really see them in action and get a feel for their dynamic nature, time lapse photography shows how the clouds continually change, and yet stay nearly in place. In the video below, you can see the lenticular clouds changing shape, but remaining relatively in place, continually forming on the upwind side and dissipating and evaporating downwind, though their forms are relatively elongated and thin and are relatively broad.
This example shows an excellent example of trains of stacked lenticular clouds hovering over occasional appearances of very turbulent rotary or roll cloud. You can see behind the first and most visually arresting set of lenticular clouds that they extend for some distance forming a wave train of multiple lenticular clouds. You can also see how small differences in the moisture content and stability of the layer of the atmosphere act to change the shape, edges, and appearances of the rotor clouds beneath them.
One of the reasons these clouds are so important for weather observers to identify is that they serve as visual indications of atmospheric turbulence, making their identification very important for aviation interests. The clouds, and the gaps between them, are indicators of upward and downward motion couplets, which if the atmospheric flow is strong, may make keeping control of aircraft, especially small aircraft, difficult if they are flown through them. Underneath lenticular clouds there is also an increased possibility of highly turbulent circular, or rotary, motion, which sometimes can be seen as ‘roll clouds’ if there is sufficient moisture in this layer. It is important to note that clouds do not form every time these atmospheric motions occur downwind of mountain ranges, so pilots should be aware that lack of these clouds does not mean that turbulence is not present!
Sources:
https://www.premierflightct.com/newsletters/TrainingArticles/StandingLenticularClouds.html
Meet Summer Interns Zakiya, Max and Maddie
Meet Summer Interns Zakiya, Max and Maddie By MWOBS Staff We are excited to welcome six teammates to the summit of Mount Washington this summer! During their internship, these students and graduates will play
Saying Goodbye to the Summit
Saying Goodbye to the Summit By Alexis George After an extraordinary last three years working as a Weather Observer and Meteorologist, I am excited to pursue a different career. As sad I as am
Top Trails for Hiking Mount Washington According to White Mountain Hiking Legends
Top Trails for Hiking Mount Washington, According to White Mountain Hiking Legends By Fawn Langerman What is the best route to hike to Mount Washington? Read on for details about the most common routes,